Hypothesis: First-Degree Inbreeding Facilitates Chromosomal Speciation
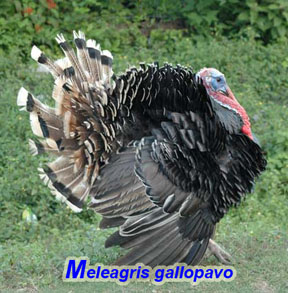
AUTHOR: Allen MacNeill
SOURCE: Original essay
COMMENTARY: That's up to you...
Happy Thanksgiving!
To help you enjoy the holiday, let me offer you a hypothesis that I have been working on to explain the origin of species in animals. The inspiration for this hypothesis was a debate at Uncommon Descent in which I have been embroiled for the past few days. The debate began with a discussion of the possibility of "virgin birth" in humans. The poster, DaveScot (not his real name) started out with a description of meiosis that contained an egregious error: that the first division of meiosis results in two diploid daughter cells. As every introductory biology student knows, this is incorrect: the first division of meiosis produces two haploid daughter cells in which the chromosomes are still double-stranded. The second division of meiosis is essentially a mitotic division, separating the sister chromatids in the double-stranded chromosomes of the first-division daughter cells.
The debate moved on, eventually centering on the subject of the chromosomal basis for speciation. I mentioned that speciation is the result of genetic isolation, and that in many cases (but not all) it is associated with chromosomal fission, fusion, inversion, and translocation events. For example, one of the main differences between humans and other great apes is that humans have one less pair of chromosomes; 46 instead of 48. Recent genomic research has shown that this difference is the result of the fusion of two of the chromosomes of great apes to form the human chromosome #2. This led to the following question from one of the participants in the debate:
"Wouldn't this fusion event have to occur within at least two members- one male, one female- of the same population in order for it to have any chance of getting passed on?"
To which I answered:
No. All that would need to happen to make this possible would be for two first-degree relatives carrying the translocation to mate and have offspring. First degree relatives (i.e. parents and offspring or full siblings) can easily have the same chromosomal mutation (i.e. a fusion/fission/translocation/inversion), as they would inherit it from a single parent. If they were to mate with each other (a not uncommon event among non-humans...and even among some humans), they would be able to produce fertile offspring carrying the same chromosomal mutation.
Yes, it is true that first degree mating carries with it the possibility of reinforcement of recessive lethal alleles. However, as many geneticists and evolutionary biologists have repeatedly pointed out, this is actually beneficial to the population within which such reinforcement happens, as the alleles are removed from the population as a result.
In other words, mating between first degree genetic relatives within a small, isolated population would have the effect of both removing deleterious alleles from the population and allowing chromosomal mutations to spread throughout the population, especially if such mutations were at all beneficial (although they would diffuse almost as well if they were selectively neutral, as would probably be the case given that no change in overall genetic information would have occurred).
Furthermore, the hypothesis that I have presented above squares very well with the currently prevailing theory of speciation: that of peripatric speciation, as first proposed by Ernst Mayr. According to Mayr's theory, speciation occurs most often in small, isolated populations on the periphery of large, panmictic populations. There is abundant natual history evidence that this is the case, especially in animals.
However, no one has yet explained how peripatric speciation would come to be associated with the kinds of chromosomal changes that we have been discussing. My hypothesis – that first-degree inbreeding facilitates chromosomal speciation – is an attempt to reconcile those two observations.
In a large, panmictic population, selection would tend to eliminate individuals who mate with first-degree relatives as a result of decreased viability due to inbreeding depression and the increased frequency of expression of homozygous lethal alleles.
However, in very small, isolated populations individuals who occasionally mate with first degree relatives (i.e. "facultative first degree inbreeders") could easily have a selective advantage of individuals who avoid mating with first degree relatives (i.e. "obligate outbreeders").
Males in particular would tend to loose less as the result of mating with first degree relatives, as their parental investment in offspring is lower (i.e. they can waste gametes and even zygotes by mating with their first degree relatives, without significantly decreasing their reproductive success).
However, even females can cut their losses by mating with first degree relatives if the likely alternative is failure to mate at all due to unavailability of non-relatives. This would especially be the case in small, isolated populations, which are exactly the kind of populations in which speciation is most likely to occur.
The effects described above would be facilitated by increased genomic homogeneity, such as would result from genetic bottlenecks and founder effects. This is because close inbreeding intensifies genomic homogeneity and decreases genetic variation, especially in isolated populations with decreased gene flow from other populations.
This hypothesis – that first degree inbreeding facilitates chromosomal speciation – immediately suggests a series of predictions, all of which are empirically testable:
• The frequency of mating between first degree relatives should be inversely correlated with effective breeding population size. That is, the smaller the effective breeding population, the greater the frequency of mating between first degree relatives (i.e. “first degree inbreeding”).
• The increased frequency of “first degree inbreeding” in such populations should be more pronounced in males. That is, males should be more likely to attempt mating with first degree relatives, especially in small, isolated populations.
• The frequency of “chromolocal mutations” (that is, chromosomal fission/fusion/inversion/translocation mutations) should also be inversely correlated with effective breeding population size. That is, the smaller the effective breeding population, the greater the frequency of viable “chromolocal mutations.”
• Peripatric speciation events should be correlated with small population size, chromolocal mutations, and first degree inbreeding.
• Speciation resulting from chromolocal mutations should be much less common in large, panmictic populations.
• First degree inbreeding should also be much less common in large, panmictic populations.
• The success rate of artificial (i.e. facilitated/forced) first degree mating should be directly correlated with the degree of inbreeding. That is, the more inbred a population, the more successful artificial first degree inbreeding should be.
• Paleogenomic analysis should find close correlations between genetic bottlenecks, founder events, and peripatric speciation events and the frequency of chromolocal mutations and genetic homogeneity (resulting from first degree inbreeding).
• Relatively large changes in phenotype resulting from chromolocal effects should be more common in small, isolated populations.
• Speciation should be easier (and therefore more frequent) among asexually reproducing eukaryotes, such as plants and parthenogenic animals (among whom aneuploidy is largely irrelevant).
Let me stress two things about the foregoing:
• What I am suggesting is, at this stage, merely a hypothesis, but one that generates a series of immediately testable predictions.
• The hypothesis is, of course, based on the idea that incest (i.e. first degree inbreeding) is the most likely explanation for the diffusion of chromolocal mutations throughout small, isolated populations of animals. Let me stress as strongly as possible that I am NOT advocating incest, I am simply pointing out that first degree inbreeding would facilitate the kind of chromolocal mutations that are often correlated with species differences in animals. The same is also true for plants, of course, but in plants we don't call it "incest," we call it "self-pollination."
I would like to also add at the end of this presentation that my reading of John Davison's papers in which he details his "semi-meiotic hypothesis" for the origin of species were an indirect inspiration for my own efforts. While his hypothesis would work, its most significant drawback is that it requires an almost unlimited number of independent "reinventions" of the same mechanism (i.e. semi-meiosis) for speciation that results from chromolocal effects to be the basis for speciation throughout the animal kingdom. Not impossible, but extremely unlikely.
By contrast, my "first degree inbreeding hypothesis" does not require independent "reinventions" of semi-meiosis at all. The only thing it requires is that first-degree inbreeding occur in small, isolated populations of animals, an easily testable prediction that does not require elaborate genetic mechanisms to produce the predicted outcome: that is, genetic isolation and subsequent speciation.
I am a little perplexed at why no one has yet proposed this mechanism, given the fact that it is already used as the explanation for speciation in plants via polyploidy. The only explanation that seems reasonable to me is that most evolutionary biologists assume that animals will always avoid mating with first-degree relatives as a result of the increased frequency of inbreeding depression and expression of homozygous lethal alleles that result from it.
Anyway, that's my hypothesis in brief. Oh, and one more thing: why the turkey at the head of this post? To commemorate Thanksgiving, of course, but also because turkeys are known to exhibit significant numbers of parthenogenesis. That is, a significant proportion of male turkeys are the result of the development of an unfertilized egg. They are male, not female (as would be the case in parthenogenetic mammals) because males are the homogametic sex in birds; they are ZZ, whereas females are ZW (the Z and W chromosomes corresponding in function to the X and Y chromosomes in mammals). It has not escaped my notice that parthenogenesis would greatly facilitate the kind of chromosomal speciation I have outlined above. Hence, the turkey can stand as an emblem of the First-Degree Inbreeding Hypothesis for Chromosomal Speciation in Animals.
Have a great turkey day, folks!
Comments, criticisms, and suggestions are warmly welcomed!
--Allen
Labels: evolution, evolutionary phylogeny, genetic variation, speciation
3 Comments:
Despite being stuffed with stuffing (not to mention roasted archosaur flesh), I have received and answered one comment on this post already. Here is the comment, and here is my answer:
Hi, Dave:
You make good points (all of which I agree with, and which also demonstrate that you understand the problem), but you miss mine. In particular, you state:
“…the only way for the fusion to become a new species is there must be another individual of the opposite sex born with the same fusion and the two individuals with the fused chromosome must find each other and mate.”
If a single individual (say a male) has a chromolocal mutation (i.e. a fission, fusion, inversion, or translocation in/of one of his chromosomes) it will generally not affect him phenotypically at all, since the same genetic information is still there, it’s just located in a different place. If he mates with someone else, he will pass his chromolocal mutation to half of his offspring on the average, as some will get the normal version of the chromosome and some will get the altered one (as the result of the segregation of the homologous chromosomes during the first division of meiosis). Again, since there is no change in genetic information, his offspring should have approximately the same fitness as himself, and so at least some of them may survive (after all, he did at least long enough to mate and reproduce). The offspring that share the chromolocal mutation can then successfully mate with either each other (or with him), as they will share the same mutation. This means that it would be unnecessary for the mutation to happen more than once, so long as the individual in which the mutation occurred can pass it on to his/her offspring.
As I pointed out, this would be more likely to happen in the case of males (who loose little or nothing from mating with someone with whom they share potentially lethal alleles), and would be more likely to happen in very small populations anyway (since alternative mates would be few and far between, or missing altogether). This squares nicely with the prevailing theory of speciation (Mayr’s peripatric theory), which states that the probability of speciation events is inversely correlated with effective breeding population size.
And so you are absolutely right in one sense: if it were necessary for two individuals who independently had the very same chromolocal mutation happen to them to find each other, it would be extremely unlikely that they would, rendering the probability of speciation as the result of chromolocal mutation almost impossible.
However, we know from simple observation that many of the differences we see in closely related species are indeed the result of chromolocal mutations. Therefore, quite apart from the question of how such mutations participate in speciation, it is necessary to explain how they can possibly get established in a population at all (barring magical intervention, of course).
My hypothesis explains how this can happen, using an extremely simple mechanism (first-degree inbreeding) and without requiring the simultaneous mutation of multiple individuals in a breeding population. It also avoids a similar problem with Davison’s hypothesis, which requires a switch from one form of gamete production to another (i.e. a fairly radical alteration of an otherwise stable genetic process) at just the right time to allow for speciation.
Davison is correct in stating that his hypothesis could be tested using amphibians, primarily because amphibian eggs can be coaxed into dividing without being fertilized. However, my hypothesis has no such limitation, and can easily be tested in virtually all animals via relatively simple observations (i.e. not requiring complex artificial manipulations under controlled laboratory conditions).
That is, my hypothesis does not require any new genetic mechanisms at all beyond chromosome fission/fusion/inversion/translocations, which we know from empirical observation are surprisingly common. All it requires is that first degree genetic relatives occasionally mate with each other, an observable and easily testable requirement (and one that artificial breeders use quite often, although for other reasons).
Furthermore, such mutations are not rare. Indeed, it is likely that every one of us acquires several of these mutations as we age, occuring in various cells throughout our bodies. Such mutations have been shown to occur regularly at a steady “background rate” in all cells. For example, at least one form of leukemia is known to be caused by a translocation of a small part of one chromosome to another, producing what is known as a “Philadelphia chromosome” (see http://en.wikipedia.org/wiki/Philadelphia_chromosome ). This is also one reason for the decline in fertility with age in most animals; they accumulate chromolocal mutations that eventually make meiosis less likely to produce viable gametes.
Also, an individual in which a chromolocal mutation has occurred will probably not be a “monster” at all. None of the genetic information carried by the chromosomes has necessarily been altered, it has simply been located somewhere else in the genome. This means that such individuals would generally be phenotypically indistinguishable from individuals who do not have such mutations.
But you are right in one sense: my hypothesis strongly implies that speciation that results from a chromolocal mutation is an event that happens in single individual, and happens essentially instantaneously. This directly contradicts mainstream evolutionary theory (i.e. the so-called “modern evolutionary synthesis”), as it strongly implies that macroevolution (i.e. speciation and the origin of higher taxa) is virtually instantaneous, rather than the long-drawn-out result of millions of years of gradual change, as implied by the “modern synthesis.”
However, this (IMNSHO) is yet another factor in favor of my hypothesis, as it squares nicely with Eldredge and Gould’s theory of “punctuated equilibrium,” which strongly suggests that macroevolution happens in relatively rapid “bursts,” followed by long periods of evolutionary “stasis.” As E&G’s theory has been steadily gaining ground, this is just great for my own hypothesis, which provides an easily testable genetic mechanism for such macroevolutionary “revolutions.”
To sum up:
As you correctly point out, the problem is having sufficient numbers of individuals sharing the same chromolocal mutation to mate and produce fertile offspring (since such individuals generally can’t successfully mate with individuals who do not share such mutations).
The solution as I see it is surprisingly simple: having individuals who share the same mutation because of common descent/parentage (i.e. full siblings or parents and offspring) mate with each other. That this would be most likely to happen in small, isolated populations squares nicely with what we know about species differences and the circumstances in which they are observed.
Why invoke an model that requires major inbreeding? You are assuming that these chromosomal rearrangements confer a fitness cost because of the rearrangements themselves. The assumption of underdominance of rearrangements is unwarranted. It's more plausible for your model to allow for rearrangement heterozygotes than to force sib mating.
However, no one has yet explained how peripatric speciation would come to be associated with the kinds of chromosomal changes that we have been discussing. My hypothesis – that first-degree inbreeding facilitates chromosomal speciation – is an attempt to reconcile those two observations.
Read this article. This is the most plausible model for speciation via genome rearrangement. The rearrangements (inversions in this case) do not cause reproductive isolation themselves, but they harbor loci responsible for the reproductive isolation between karyotypes.
For more on this hypothesis of chromosomal speciation, check out the work of Noor, Rieseberg, and Feder.
One other point: the mechanism that I have proposed (i.e. first degree inbreeding) for the establishment of viable populations of individuals with chromolocal mutations would apply much more easily to most other eukaryotes, especially plants, most of which can easily self-cross. This would imply that speciation would be easier among plants, and indeed might be so easy as to invalidate the entire concept of "species" among plants.
Which was the point of my post on "The Origin of the Specious" (see http://evolutionlist.blogspot.com/2006/03/origin-of-specious.html)
Post a Comment
<< Home